Friday Mar. 30, 2018
Blind Boys of Alabama on this Good Friday "Way Down in
the Hole" (3:22), "If I Had a
Hammer" (4:45), "Amazing Grace"
(4:51), "I
Shall Not Be Moved" (4:28), "Soldier"
(3:30)
Precipitation-producing processes
Fortunately there are two processes capable of quickly turning
small cloud droplets into much larger precipitation particles in a
cloud.
The collision coalescence process works in clouds that are
composed of water droplets only. We'll
see that this is a pretty easy process to understand.
This is often called the "warm rain"
process. Clouds like this are found in the tropics (and very
occasionally in Tucson). Because warm air can contain much
more moisture than colder air, the "warm rain" process is capable
of producing very heavy rainfall.
This process will only produce rain, drizzle, and something
called virga (rain that evaporates before reaching the
ground). Because the clouds are warm and warm air can
potentially contain more water vapor than cooler air, the
collision-coalescence process can produce very large amounts of
rain.
The ice crystal process produces precipitation everywhere
else. This is the process that normally makes rain in
Tucson, even on the hottest day in the summer (summer thunderstorm
clouds are tall and reach into cold parts of the atmosphere, well
below freezing). Hail
and graupel often fall from these
summer storms; proof that the precipitation started out as an ice
particle). Thunderstorms also produce lightning and later in
the semester we will find that ice is
needed to make the electrical charge that leads to lightning.
There is one part of this process that is a
little harder to understand, but look at the variety of
different kinds of precipitation particles (rain, snow,
hail, sleet, graupel, etc) that can result.
Here's how the collision coalescence process works. The
picture below shows what you might see if you looked
inside a warm cloud with just water droplets:
The collision coalescence
process works in a cloud filled with cloud droplets of
different sizes, that's critical. The larger
droplets fall faster than the small droplets. A
larger-than-average cloud droplet will overtake and collide with
smaller slower moving ones.
The bigger droplets
fall faster than the slower ones. They collide and
stick together (coalesce). The big drops gets even
bigger, fall faster, and collide more often with the smaller
droplets. This is an accelerating growth process -
think of a growing ball of snow as it rolls down a
snow-covered hill and picks up snow, grows, and starts to
roll faster and faster; or think of an avalanche
that gets bigger and moves faster as it travels downslope.
Very quickly a larger than average cloud droplet can grow
to raindrop size.
The figure shows the two precipitation producing clouds:
nimbostratus (Ns) and cumulonimbus (Cb). Ns
clouds are thinner and have weaker updrafts than Cb clouds.
The largest raindrops fall from Cb clouds because the droplets
spend more time in the cloud growing. In a Cb cloud raindrops can
grow while being carried upward by the updraft (in this case the
smaller droplets are catching and colliding with the larger
droplets, but the end result is the same) and also when falling in
the downdraft.
Raindrops grow up to about 1/4 inch in diameter. When
drops get larger than that, wind resistance flattens out the drop
as it falls toward the ground. The drop quicly breaks apart
into several smaller droplets. Solid precipitation particles
such as hail can get much larger (an inch or two or three in
diameter).
And actually my sketch at lower left above isn't quite accurate
as this video of the breakup of a
5 mm diameter drop of water shows.
The ice crystal process works in most locations most of the
time. Before we can look at how the ice crystal process
actually works we need to learn a little bit about clouds that
contain ice crystals - cold clouds.
Cold clouds
The figure below shows the interior of a
cold cloud.
The bottom of the thunderstorm, Point 1, is warm enough (warmer
than freezing) to just contain water droplets. The top of
the thunderstorm, Point 2, is colder than -40 F (which,
coincidentally, is equal to -40 C) and just contains ice
crystals. The interesting part of the thunderstorm and the
nimbostratus cloud is the middle part, Point 3, that contains both
supercooled water droplets (water that has been cooled to below
freezing but hasn't frozen) and ice crystals. This is called
the mixed phase region.
This is where the ice crystal process will be able to produce
precipitation. This is also where the electrical charge that
results in lightning is created.
Ice crystal nuclei
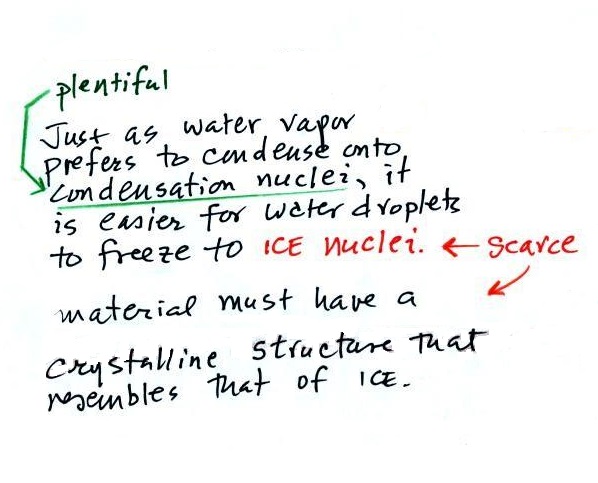
The supercooled water droplets aren't able
to freeze even though they have been cooled below
freezing. This is because it is much easier for small
droplets of water to freeze onto an ice crystal nucleus (just
like it is easier for water vapor to condense onto
condensation nuclei rather than condensing and forming a small
droplet of pure water). Not just any material will work
as an ice nucleus however. The material must have a
crystalline structure that is like that of ice. There
just aren't very many materials with this property and as a
result ice crystal nuclei are rather scarce. In most of
the mixed phase region there are more supercooled water
droplets than ice crystals.
Supercooled water
Here are a couple of demonstrations involving supercooled water
that I showed in class. In the first
demonstration, some supercooled water (cooled to -6 F (-21
C)) is poured into a glass bowl sitting at room
temperature. Just pouring the water into the bowl is
enough of a "disturbance" to cause the supercooled water to
freeze. Just bumping a bottle of supercooled water in the second
video is enough to cause the water to freeze. I have
no idea why bumping the bottle of water initiates freezing.
Superheated water
It is also possible to superheat water.
When the superheated water is disturbed it
suddenly and explosively begins to boil. This
is a potentially dangerous demonstration to attempt, better to
watch a
video online.
Here are a some precautions just in case you're ever tempted to
try an experiment like this.
It is probably easier to superheat distilled water than ordinary
tap water. So you might put two cups of water into a
microwave, one with tap water the other filled with distilled
water. The cup of tap water will probably start boiling when
it is supposed to, i.e. before it can become superheated.
You can watch the tap water and get an idea of how long you need
to heat the distilled water to superheat it. I suspect
impurities in the tap water might act as nuclei to initiate the
boiling.
Then once you think you have superheated the cup of distilled
water be very careful taking it out of the microwave (better yet
leave it in the microwave). Just the slightest disturbance
might start the water boiling. You want your hands, arm,
body and faced covered and protected just in case this
happens. Tape a spoon onto the end of a long stick and put a
little sugar or salt into the spoon. Then drop the salt or
sugar into the cup of superheated water.
Chemists will often use "boiling chips" to make sure water will
start to boil when it is supposed to (at 212 F) rather than
becoming superheated.
Bubbles in beer or soda
Rather than superheating water, here's a far safer
experiment to try.
Carbonated drinks all contain dissolved carbon dioxide. The
drink containers are pressurized. When you open the can or
take the cap off the pressure inside is released and dissolved
carbon dioxide gas starts to come out of solution and forms small
bubbles. Often you will see the bubbles originate at a point
on the side or bottom of the glass. These are nucleation
sites and are often small scratches or pits on the surface of the
glass that are filled with a small bubble of air. You can
think of these bubbles of air as being "bubble nuclei." When
the carbon dioxide comes out of solution rather than forming a
small bubble of its own, it makes use of and builds on these
existing bubbles of air. The bubble, now a mixture of air
and carbon dioxide, grows until it is able to break free and float
to the surface (a little gas is left behind in the scratch so the
process can start over again).
This is actually a michelada, I think; a
mixture of beer, lime, and tomato juice (image
source). The juices don't affect the bubble
formation
The next time you are drinking one of these carbonated beverages
sprinkle in a few grains of sugar or salt. These will serve
as additional bubble nucleation sites and additional bubbles will
form. This is exactly what happened in the superheated water
demonstration above.
I'm not sure whether there will be
time to get to this next section on Friday, but I've included
it just in case
Next- the "tricky" part of the Ice Crystal Process:
what actually gets precipitation formation started
We'll see next why or how the
ice crystal process works, this is the "tricky" part. It's
a 3-step process (summarized on p. 101 in the ClassNotes)
The first figure above shows a water droplet in equilibrium with
its surroundings. The droplet is evaporating (the 3 blue
arrows in the figure). The rate of evaporation will depend
on the temperature of the water droplet. There will be some
evaporation even from a droplet that is very cold. The
droplet is surrounded by air that is saturated with water vapor
(the droplet is inside a cloud where the relative humidity is
100%). This means there is enough water vapor to be able to
supply 3 arrows of condensation. Because the droplet loses
and gains water vapor at equal rates it doesn't grow or shrink.
This figure shows what is
required for an ice crystal (at the same temperature) to be in
equilibrium with its surroundings. First, the ice crystal
won't evaporate as rapidly as the water droplet (only 1 arrow is
shown). Going from ice to water vapor is a bigger "jump"
than going from water to water vapor. There won't be as
many ice molecules with enough energy to make that jump. A
sort of analogous situation is shown in the figure below.
The class instructor could and most of the people in the room
could jump from the floor to the top of a 10 or 12 inch tall
box. It would be much tougher to jump to the top of the
table (maybe 30 inches off the ground) or the cabinet (maybe 36
inches) at the front of the room. There wouldn't be as
many people able to do that.
To be in equilibrium the ice crystal only needs 1 arrow of
condensation. There doesn't need to be as much water vapor
in the air surrounding the ice crystal to supply this lower rate
of condensation.
Sublimation (solid to gas) is a bigger jump than evaporation
(liquid to gas). Not as many ice molecules are able to make
the big jump as there are making the smaller jump
Now what happens in the mixed phase region of
a cold cloud is that ice crystals find themselves in the very
moist surroundings needed for water droplet equilibrium. This
is shown below.
The water droplet is in equilibrium (3 arrows of evaporation and
3 arrows of condensation) with the surroundings. The ice
crystal is evaporating more slowly than the water droplet.
But the ice crystal is in the same surroundings as the water
droplet and water vapor will be condensing onto the ice crystal
at the same rate as onto the water droplet. The ice crystal isn't in
equilibrium, condensation (3 arrows) exceeds evaporation (1
arrow) and the ice crystal will grow. That's part
of what makes the ice crystal process work.
The equal rates of condensation are shown in the figure
below using the earlier analogy.