You
could
walk by the house late in the
afternoon, when the students might
be outside, and count them.
That would be a direct
measurement. There could still be
some errors in your measurement
(some students might be inside the
house and might not be counted,
some of the people outside might
not actually live at the house).
If you were to walk by early in
the morning it is likely that the
students would be inside
sleeping. In that case you
might look for other clues (such
as the size of the house, or the
number of automobiles or bicycles
parked in the yard) that might
give you an idea of how many
students lived there. Some
calibration would be needed but
you could use this other
information to estimate the number
of students present.
3. Paleoclimatology
Scientists have
come up with a variety of
ingenious ways of determining past
temperature and climate. Several
of them are shown in
the figure
below. Pay
careful attention to
the logarithmic time
scale at the bottom of
the figure. It
probably should have
been labeled time
before the
present. The
present day is shown
at right and is marked
zero.
We'll work our way from the bottom
to the top of the chart and
briefly look at a few examples.
3a.
Geological evidence
Earlier in the course we saw how
the banded iron rock formation
provides evidence of the early
buildup of oxygen in the
oceans. The oxygen was
produced by blue-green algae using
photosynthesis at a time (roughly
2 to 3 billion years ago) when
there was little or no oxygen in
the earth's
atmosphere. We may
also have mentioned red beds,
sedimentary rock that has a rusty
color because it contains iron
oxides. These red beds
are evidence of oxygen buildup in
the atmosphere. The
photograph below from Red Rock
State Park near Sedona (in
northern Arizona) shows Permian
red beds (source
of this image).
The Permian period was roughly 250
to 300 million years ago.
There are red beds older than that
but not more than 2 billion years
old.
Rocks and geological formations
can tell us a lot about the
distant past. The earth has
been alternating between glacial
and interglacial periods for the
past 1 to 2 million years (more
about this in Part 3 of this
series). Evidence of
glacial periods is often preserved
in rock.
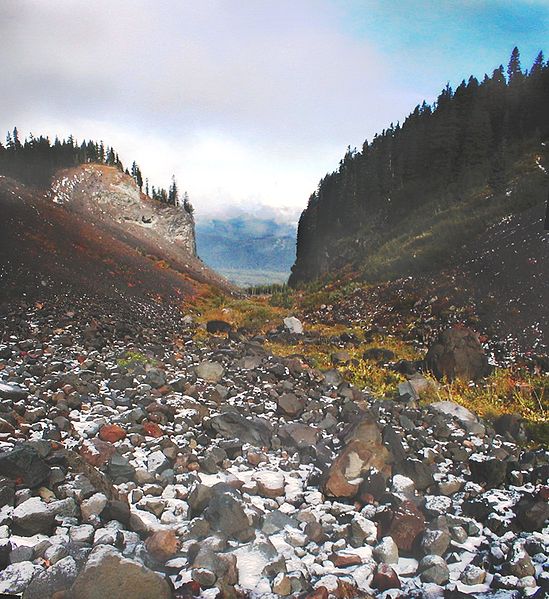
|
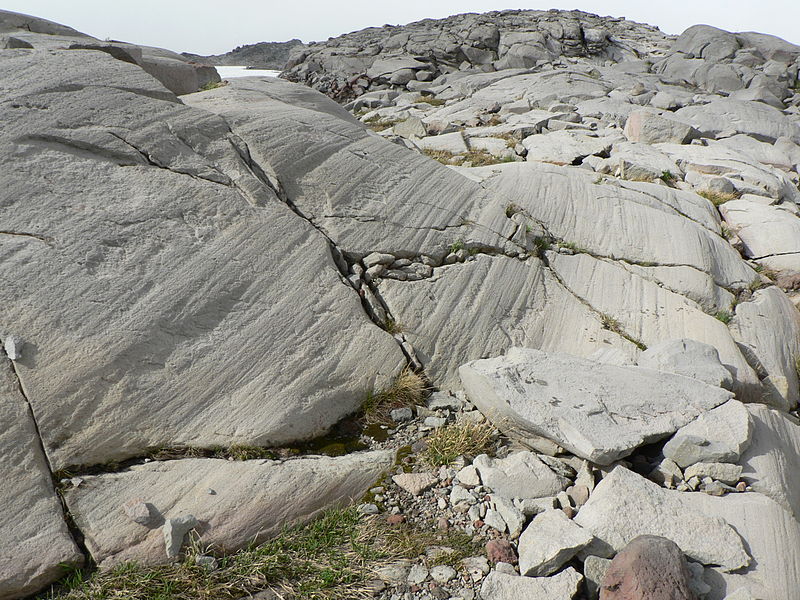
|
A U-shaped valley
carved out by a relatively small
glacier in the Mt. Hood Wilderness (source of this
photograph)
|
Glacial striations
found in the Mt. Rainier National
Park (source
of this image)
|
U-shaped valleys with steep sides
and a relatively flat bottoms are
produced by the scouring action of
glaciers moving downhill.
The grinding motion of the ice
against stone will often leave
clearly visible striations.
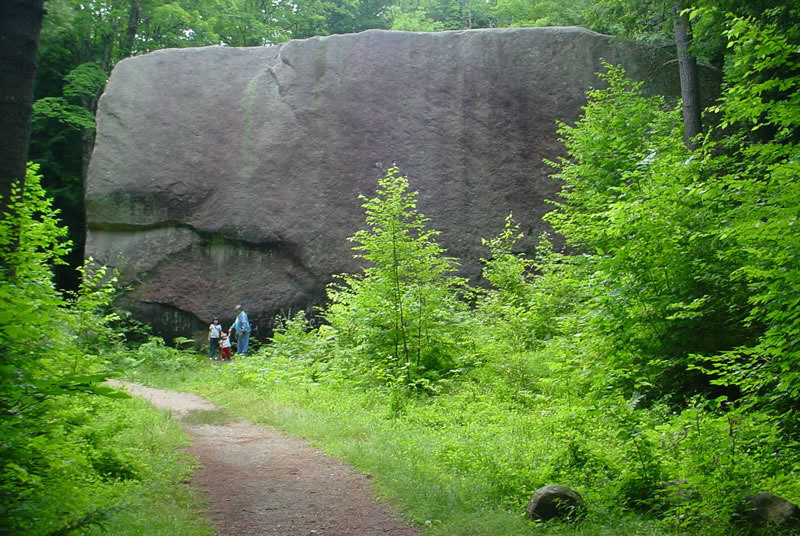
|
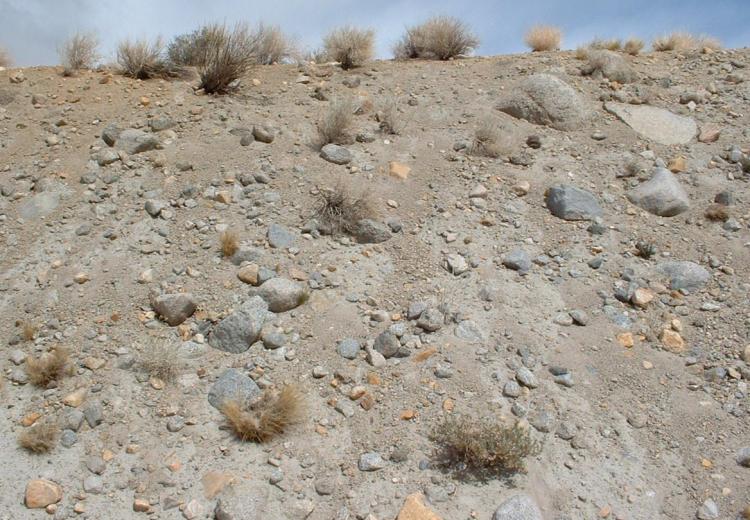
|
A photograph of the
Madison Boulder in New Hampshire
(from Atlas
Obscura).
|
Glacial till
(produced and moved around by a
glacier) exposed in a road cut (source
of this photograph)
|
An erratic (also called a
dropstone) is a large rock or
boulder carried by a glacier and
then dropped when the ice
melted. Erratics are often
found quite far from their place
of origin and often in a region
with completely different types of
soil and rock.
The
12 million pound Madison Boulder
shown above is the largest erratic
in New England and one of the
largest in the world.
Plymouth Rock, near where the
Mayflower landed in 1620, is
apparently also a glacial erratic
(it has been moved from its
original location to a more
protected site because of its
historical significance).
Dropstones can also be carried by
icebergs and fall to the sea floor
when the iceberg melts.
Stones in Erratic
Rock State Natural Site in
Oregon were carried more than 500
miles during ice age flooding of
the Columbia River.
Glaciers
erode the surface
over which they
move and produce
sediment, "till",
that is an
unsorted mix of
many particle or
rock sizes.
3b. Ocean
and lake sediments
Life forms in ocean fall to the
ocean floor when they die.
Sea floor sediments build up year
after year and are probably the
longest continuous record of
climate change that is available.
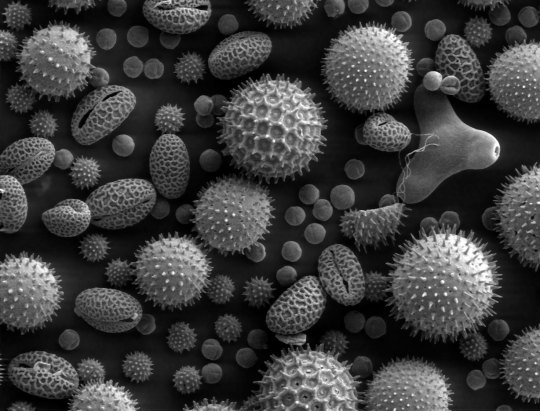
|
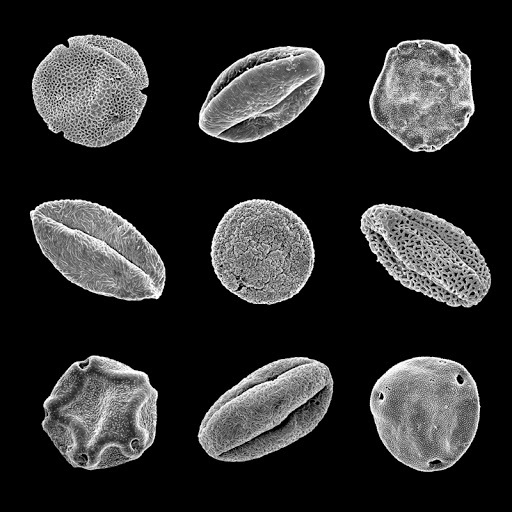
|
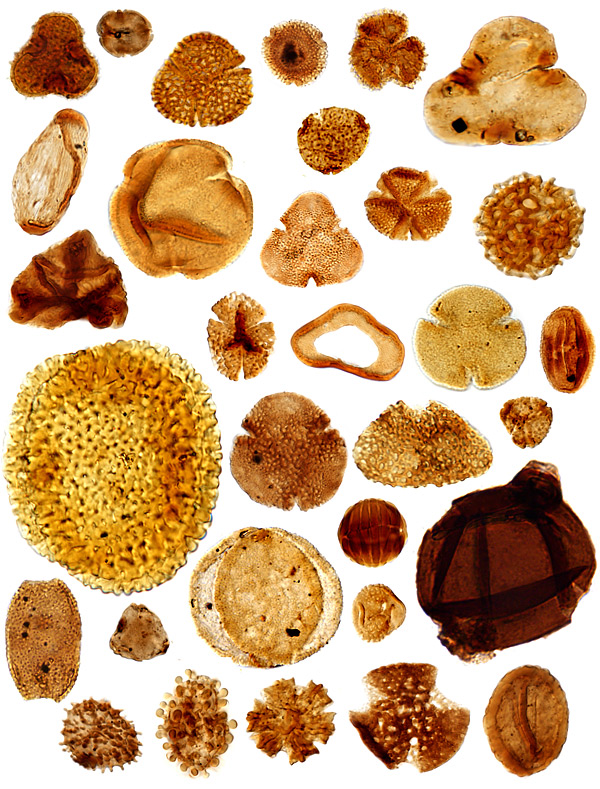
|
Scanning electron
microscope photograph of grains of
(unfossilized) pollen. (source)
|
Fossilized pollen
from China. (source)
|
Electron microscope
photographs of fossilized pollen
from the Paleocene-Eocene Thermal
Maximum 56.3 million years ago. (source)
|
Plant pollen and spores are
abundant and particularly well
preserved in core samples from
lake beds and the sea floor.
With care they can be used to
infer past environmental and
climatic conditions. Size
scales weren't included in the
figures above, but the examples
shown are probably 10 to a few 10s
of micrometers (millionths of a
meter) across.
Identification (and dating) of the
types of pollen present provides
information about the
environmental conditions at the
time.
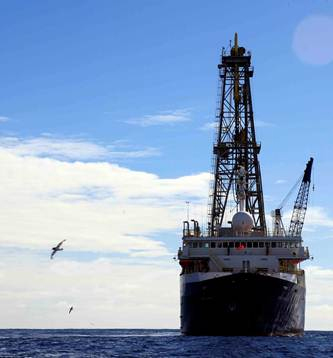
|
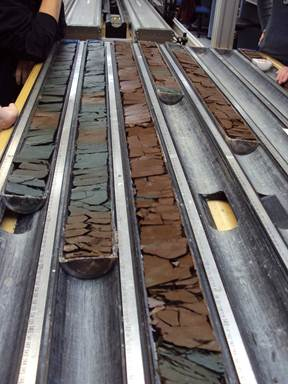
|
The drill ship JOIDES
Resolution located off the
coast of Antarctica in Spring
2010. Researchers were able to
drill 1000 m below the sea floor and
obtained sediment samples that were
over 50 million years old.
(photo credit: Rob Dunbar Stanford
University
|
Photographs of core
samples obtained during the
expedition. Pollen and spores
were preserved in the marine
sediment. (photo credit: Kevin Welsh
University of Queensland)
|
The photographs above are from an
online article titled "Tropical
rainforest on the South Pole"
from Ultrecht University in the
Netherlands.
3c. Tree
rings
Tree rings (dendrochronology)
provide another way of determine
recent changes in climate.
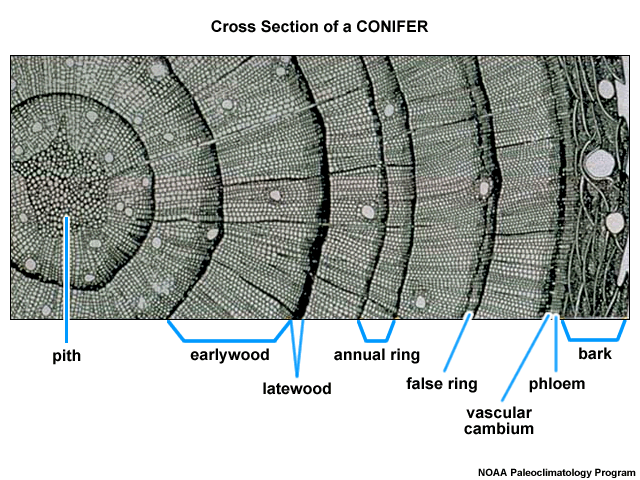
|
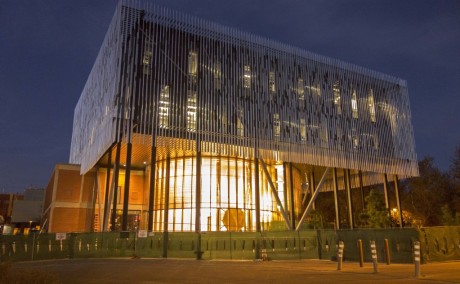
|
Tree rings form
because of changes in the rate of
growth of a tree during the
year. Light colored low
density wood forms early in the
year. A thinner, darker, more
dense band forms late in the
year. (source
of this image)
|
The Laboratory
of Tree Ring Research at the
University of Arizona recently moved
into this new building. Their
offices and laboratories used to be
located in the football stadium.
|
The date of each ring can be
determined. The widths of
the rings are determined by
temperature and precipitation
during the year of that growth
(and other factors).
Bristlecone pine trees in the
United States provide a continuous
record of climate change that
extends back about 8500
years. A record this long
does not come, of course, from a
single tree.
Rather part of the pattern of
rings in a living tree can be
matched to a portion of the of
rings in an older, dead tree and
used to date that tree. The
dead tree record which now extends
further back in time than the
living tree can be used to date a
third tree and so on further and
further back in time. Or, as
shown in the figure above, cross
matching between several wood
samples can be used to date the
wood in an old structure of
historical interest.
3d.
Historical documents
Historical records such as time
and types of crops that were
planted and whether the ensuring
harvest was good or bad;
exceptional periods of cold or
heat, droughts, snow or storm
damage provide a qualitative idea
of the climate at the time.
From written accounts of Norse
sagas (and much later
archaeological work) we know that
Vikings settled Iceland,
Greenland, and parts of
Newfoundland during a warm period
that lasted from about AD 800 to
AD 1300. Iceland was
apparently settled between about
874 AD and 930 AD. One
of the early settlers, Erik the
Red, was banished from Iceland
(for manslaughter) and is credited
with establishing the first colony
on Greenland in about 985
AD. Settlements on Greenland
lasted nearly 500 years, until the
climate began to cool. The
total population reached several
1000 people. Leif Erikson,
the son of Erik the Red, is often
credited with the establishment of
the first Norse settlement on
North America (at L'Anse aux
Meadows in Newfoundland in about
1000 AD).
Recreation
of a sod longhouse at L'Anse aux
Meadows, Newfoundland (source of
this image)
3e.
Isotopic analyses
Core samples from ice sheets in Greenland
and Antarctica were used to determine
atmospheric carbon dioxide concentrations
in the past. The ice can also be
used to determine temperature. The
basic idea is to determine the relative
amounts of oxygen isotopes in the H2O that
makes up ice.
The
two
isotopes of oxygen contain
different numbers of neutrons in
their nuclei. Both
atoms have the same number of
protons.
During
a cold period, the somewhat
lighter H2O16
form of water evaporates more
rapidly than H2O18.
You would find relatively
large amounts of O16
in glacial ice. Since most
of the H2O18 remains
in the ocean, it is found in
relatively high amounts in calcium
carbonate in ocean
sediments. Note
also
the drop in ocean levels during
colder periods when much of the
ocean water is found in ice sheets
on land.
The
reverse is true during warmer
periods.
Coral can also be
analyzed. Coral is made
up of calcium carbonate, a
molecule that contains
oxygen. The relative
amounts of the oxygen-16 and
oxygen-18 isotopes depends on
the temperature that existed
at the time the coral
grew.
4. 2000 year long temperature
record
Using proxy
data scientists have been able
to estimate average surface
temperatures for 100,000s of
years into the past. The
next figure shows what
temperature has been doing
since 1000 AD. This is
for the northern hemisphere
only, not the globe.
The
green
shaded portion of the
graph shows the estimates
of temperature (again
relative to the 1961-1990
mean) derived from proxy
data. The red shaded
portion shows the
instrumental measurements
were made between about
1850 and the present
day.
The figure above just
shows the overall trend in
temperatures during the
past 1000 years. The
actual data that the curve
above is based on is shown
below.
This is the so called "Hockey
Stick Plot" originally published
in 1999 by Mann,
Bradley, and Hughes (and
included in Climate
Change
2001 - The Scientific Basis,
Contribution of Working Group I to
the 3rd Assessment Report of the
Intergovernmental Panel on
Climate Change (IPCC)).
Many scientists would
argue that this graph is
strong support of a
connection between rising
atmospheric greenhouse gas
concentrations and recent
global warming.
Early in this time
interval when CO2
concentration was
constant, there are only
modest changes in
temperature. The
largest overall change in
temperature begins in
about 1900 when we know an
increase in atmospheric
carbon dioxide
concentrations was
underway. The second
half of the 20th century
is the warmest period in
at least the past 1000
years.
Some scientists have
questioned the statistical
methods used in the
study. Additionally
there is historical
evidence in Europe of a
medieval warm period
lasting from 800 AD to -
1300 AD or so and a cold
period, the "Little Ice
Age, " which lasted from
about 1400 AD to the mid
1800s. These are
naturally caused changes
in climate that are not
clearly apparent in the
temperature plot
above. This leads
some scientists to
question the validity of
this temperature
reconstruction.
Scientists also suggest
that if large changes in
climate such as the
Medieval warm period and
the Little Ice Age can
occur naturally, then
maybe the warming that is
occurring at the present
time also has a natural
cause. There is also
some question whether
these were
regional, not
global, events.
The so-called Year
Without a Summer is
another example of a
sudden short lived change
in climate. It
occurred in 1816, toward
the end of the Little Ice
Age. The unusually
cold summer temperatures
were apparently caused by
a very large volcanic
eruption the year
before. Here's
a
short
explanation
of
how
volcanoes
can
cause
short term cooling.
More
recent temperature
reconstructions have
confirmed the overall
trend shown in the figure
above.
This
is from the University of East
Anglia Climatic Research
Unit again.
The figure below shows results
from 10 different research
studies and extends the
temperature reconstruction
back 2000 years.
This
figure was created
for Global
Warming Art
by Robert
A. Rohde and
is discussed
further here.
Somewhat
larger temperature
variations associated with
the Medieval Warm Period
and the Little Ice Age can
be seen in this and the
preceding figure. In
both figures again the
late 20th century has the
warmest temperatures in
the period.
We'll end this installment
at this point.
Here's a brief summary of
where we stand:
There
is general agreement that
atmospheric CO2
and other greenhouse gas
concentrations are increasing
and that the earth
is warming.
Not everyone agrees on
the causes
(natural or manmade) of the
warming.
At times in the past the earth
has been much warmer and also
much colder than it is
now. These changes all
have natural causes.
Before looking at how man
might presently be causing
climate to change we need to
have a look at the climate
history of the earth.
That will be the topic of the
next section in this series.